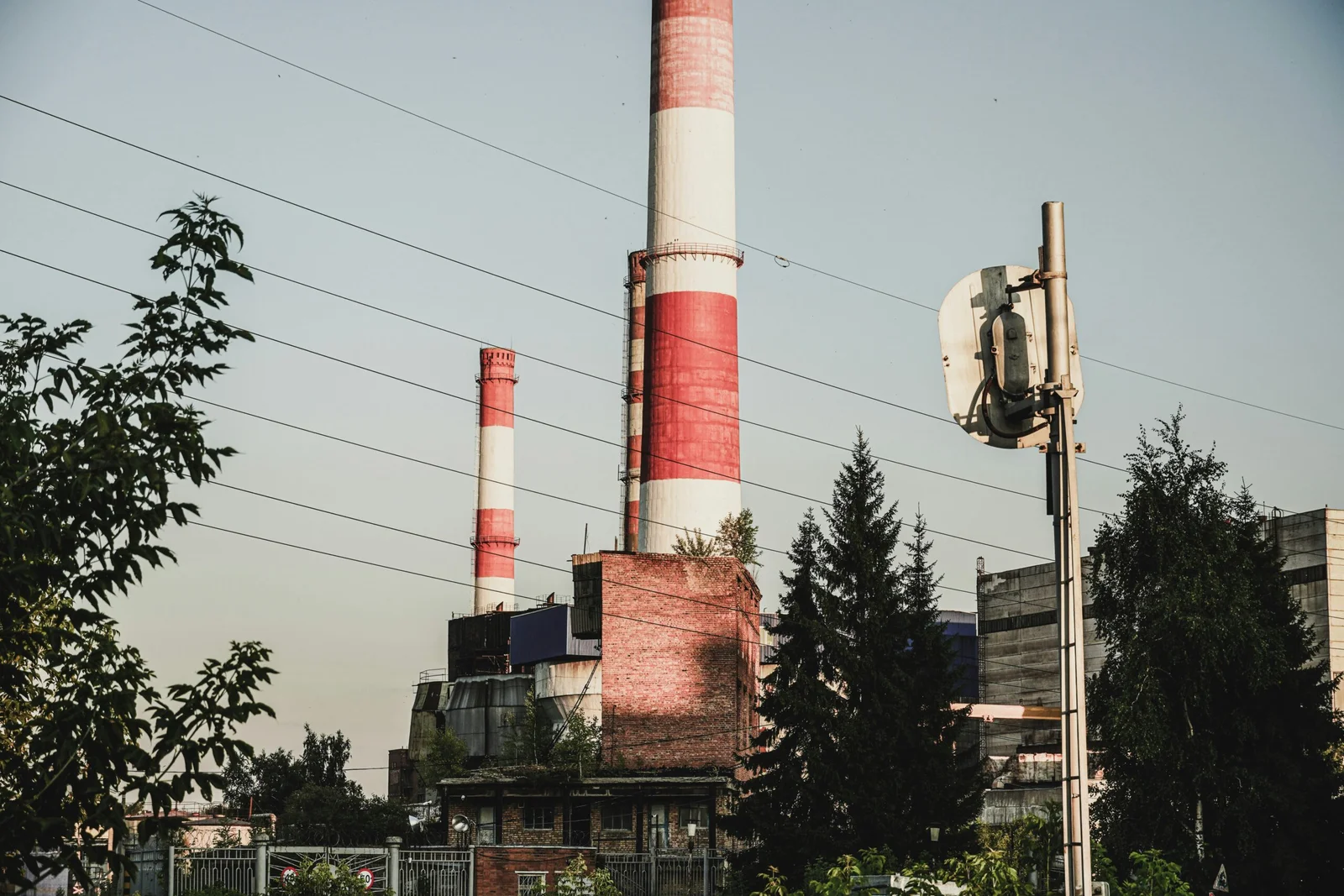
Understanding Steam Turbines: The Backbone of Energy Production
Introduction to Steam Turbines
Steam turbines are a fundamental component in the generation of electricity within the energy sector. They operate on principles derived from thermodynamics and mechanics, converting thermal energy contained in steam into mechanical energy. This mechanical energy is then transformed into electrical energy through generators, making steam turbines a pivotal link between various forms of energy production and the electricity grid.
The operating principle of a steam turbine is relatively straightforward. Water is heated in a boiler, creating steam at high pressures and temperatures. This steam then flows into the turbine, where it expands and spins blades connected to a rotor. As the turbine blades rotate, they transfer energy to the rotor, which, in turn, drives the generator to produce electrical energy. The efficiency of this conversion process can significantly impact the overall output and operational costs of power plants.
Steam turbines have a long history, developing alongside advancements in engineering and technology. The first significant break in steam turbine technology came in the late 19th century with the invention of the reaction turbine byCharles Parsons. This innovation allowed for a more efficient transformation of steam energy compared to previous steam engines, setting the stage for the widespread adoption of steam turbines in power generation. Since then, the designs have evolved, with modern turbines operating at higher pressures and temperatures, thus achieving greater efficiency and output.
Today, steam turbines are indispensable in conventional power plants, as well as in renewable energy systems such as concentrated solar power and biomass plants. Their ability to efficiently convert thermal energy into electrical energy makes them central to sustainable energy solutions, underlining their importance in our efforts to meet growing energy demands while adhering to environmental standards.
The Working Principle of Steam Turbines
The steam turbine operates based on one of the fundamental principles of thermodynamics, specifically harnessing the energy generated from steam expansion. The process begins with steam generation, which typically occurs in a boiler where water is heated to reach a high-temperature state. This steam, under high pressure, becomes the primary working fluid for the turbine system. The thermodynamic cycle that governs this process is generally the Rankine cycle, which consists of four essential stages: heating, expansion, condensation, and pumping.
Components of a Steam Turbine
A steam turbine is a complex machine composed of several critical components that work together to convert energy stored in steam into mechanical energy, which can be further transformed into electrical energy. Understanding these components is essential for appreciating the efficiency and functionality of steam turbines in energy production.
One of the most vital parts of a steam turbine is the rotor. The rotor is the rotating element of the turbine, which includes the shaft and attached blades. As high-pressure steam enters the turbine, it impinges on the blades mounted around the rotor, causing it to spin. The design of the rotor, including its size and weight, directly influences the turbine’s operating efficiency.
The blades themselves are also crucial components. They are optimized for aerodynamics to ensure that steam flow is converted into rotational energy as efficiently as possible. The blades’ configuration can vary depending on the specific type of turbine, but they are generally designed to handle high-pressure conditions and provide maximum thrust, thereby enhancing overall turbine performance.
Another key component is the casing. The casing encases the rotor and blades, providing structural support and maintaining a controlled environment for the high-pressure steam. It serves to direct the steam flow properly and help contain the steam’s pressure, which is critical for maintaining efficiency during operation.
Lastly, the condensing system is an integral part of the steam turbine setup. After steam passes through the turbine, it is typically directed to a condenser where it loses heat and transforms back into water. This process is essential for maintaining a closed loop in the steam cycle, ensuring that the same water can be reheated and reused multiple times, thus optimizing energy efficiency.
Types of Steam Turbines
Steam turbines play a crucial role in energy production, serving various applications across the industry. Understanding the different types of steam turbines is essential for grasping their functionality and suitability for specific conditions. The most common classifications of steam turbines include impulse turbines, reaction turbines, and back-pressure turbines, each with unique characteristics and applications.
Impulse turbines operate on the principle of converting thermal energy into mechanical energy through high-speed jets of steam. In these turbines, steam is directed through nozzles that increase its velocity before hitting the turbine blades. This design allows for a simple construction and robust efficiency, making impulse turbines well-suited for power plants where high-speed operation is required. A prevalent example of this type is the Pelton wheel, often used in hydroelectric power stations to harness water energy.
In contrast, reaction turbines generate power through a combination of impulse and reaction forces, with pressure and velocity induction taking place in the rotor. As steam passes through stationary blades and then moves onto moving blades, it expands, contributing to a pressure drop that drives the turbine. Reaction turbines are commonly utilized in larger power plants and offer higher efficiency over a range of loads, which makes them versatile in electricity generation. The most notable examples include the Parsons turbine, frequently seen in various industrial applications.
Back-pressure turbines function differently, as they allow steam to exit the turbine at a pressure sufficient for thermal utilization in processes such as heating. This type of turbine efficiently combines power generation with the provision of steam for industrial applications, achieving both electricity and productive steam. Such versatility makes back-pressure turbines essential in industries like chemical processing and district heating systems.
In summary, understanding the distinctions among impulse turbines, reaction turbines, and back-pressure turbines highlights their specific roles in energy production, thereby facilitating more effective energy strategies across diverse applications.
Efficiency and Performance of Steam Turbines
Steam turbines are a fundamental component in energy production, converting thermal energy from steam into mechanical energy. The efficiency of a steam turbine system is primarily influenced by various design and operating factors. Understanding these factors is crucial for optimizing performance in power generation applications.
Thermal efficiency refers to the ratio of useful work output to the heat input. In steam turbines, thermal efficiency is significantly affected by the temperature and pressure of the steam entering the turbine. Higher steam conditions generally result in greater thermal efficiency, enabling the turbine to extract more energy from the steam. The Rankine cycle, which forms the basis of most steam turbine operations, indicates that optimizing the cycle’s parameters leads to improved energy conversion rates.
Mechanical efficiency, on the other hand, pertains to the turbine’s ability to convert mechanical energy into usable energy for generating electricity. Factors affecting mechanical efficiency encompass frictional losses, bearing performance, and the design of the turbine blades. Advanced blade geometries and materials have been developed to minimize drag and optimize flow, enhancing overall mechanical performance. Additionally, maintenance practices that ensure optimal operating conditions can significantly impact the mechanical efficiency of steam turbines.
Recent technological advancements have played a pivotal role in boosting the efficiency of steam turbines. For instance, the integration of digital monitoring and control systems enables real-time adjustments to operating conditions, enhancing both thermal and mechanical efficiencies. Furthermore, innovations such as combined cycle power plants, which utilize both gas and steam turbines, exemplify how hybrid systems can maximize energy production. These developments illustrate a growing commitment to sustainable energy practices while increasing the overall efficiency and performance of steam turbine systems.
Applications of Steam Turbines
Steam turbines play a crucial role in various sectors, primarily within the energy production industry, but their applications extend beyond electricity generation. One of the most significant areas where steam turbines are utilized is in power plants, specifically those designed for large-scale electricity generation. These high-efficiency turbines convert thermal energy from steam into mechanical work, which drives generators to produce electricity. For example, modern combined cycle power plants leverage steam turbines in conjunction with gas turbines to achieve greater efficiency by capturing waste heat from the gas turbine exhaust to produce steam for the steam turbine cycle.
In addition to electricity generation, steam turbines are also employed in marine propulsion systems. They are favored for their ability to generate high power outputs while maintaining relatively compact sizes compared to alternative propulsion technologies. The use of steam turbines in naval ships is a well-documented instance. For example, some aircraft carriers and submarines utilize steam propulsion systems, as they allow for significant speeds over extended distances, which is ideal for military applications and commercial shipping vessels.
Moreover, steam turbines find applications in various mechanical drive systems across diverse industrial sectors. Industries such as oil and gas, chemical, and manufacturing harness steam turbines to provide the drive required for pumps, compressors, and other mechanical equipment. By integrating steam turbines into these processes, manufacturers can achieve enhanced efficiency and reduce operational costs. A notable example includes refineries that pair steam turbines with process steam to drive heavy machinery, thereby boosting productivity.
Overall, the versatility of steam turbines speaks to their vital role in energy production, marine propulsion, and industrial applications, making them a backbone technology in today’s energy and industrial landscapes.
Challenges and Innovations in Steam Turbine Technology
The steam turbine industry faces a myriad of challenges that significantly affect its operational efficiency and longevity. One of the primary concerns is wear and corrosion, which can lead to decreased performance and increased maintenance costs. The high temperatures and pressures associated with steam turbine operations create an environment conducive to material degradation. Consequently, developing materials that can withstand these harsh conditions is imperative for ensuring the reliability and efficiency of steam turbines. Innovations in advanced materials, such as nickel-based superalloys and ceramic composites, are being explored to mitigate these issues and enhance the overall lifespan of these machines.
Moreover, operational efficiency remains a critical focus as the industry strives to meet growing energy demands while minimizing consumption. Conventional steam turbines often encounter inefficiencies that can lead to energy losses. To tackle this, emerging technologies such as high-efficiency turbine designs and sophisticated control systems are being adopted to optimize performance. For instance, integrated digital controls can facilitate real-time performance monitoring, allowing operators to make informed adjustments that improve efficiency and reduce fuel consumption.
Environmental impact is another key challenge that cannot be overlooked. Steam turbines, particularly those powered by fossil fuels, contribute to greenhouse gas emissions, raising concerns about climate change and sustainable energy production. In response, the industry is actively pursuing hybrid energy systems that combine steam turbines with renewable energy sources. This approach not only enhances sustainability but also diversifies energy portfolios, ultimately contributing to a more resilient energy infrastructure.
In conclusion, while the steam turbine industry grapples with significant challenges such as wear, operational inefficiency, and environmental concerns, ongoing innovations in materials and technology hold promise for the future. By leveraging advanced solutions, the industry can enhance the performance and sustainability of steam turbines, thereby securing their role as a cornerstone in energy production.
Future of Steam Turbines in Energy Production
The future of steam turbines within the energy sector remains a topic of significant interest, particularly as various trends influence their design and operation. One primary consideration is the ongoing integration of renewable energy sources into the traditional power generation mix. Steam turbines are expected to play a crucial role in hybrid energy systems that combine fossil fuels and renewable inputs. This integration allows for a more reliable and flexible power supply, meeting demand during peaks while effectively utilizing fluctuating renewable sources, such as solar and wind.
As nations around the globe strive toward ambitious carbon reduction goals, steam turbines are evolving to enhance their efficiency and minimize environmental impacts. Modern developments in turbine technology, such as advanced materials and innovative designs, aim to increase thermal efficiency and decrease emissions. Consequently, steam turbines can adapt to utilize cleaner fuels, including biomass and hydrogen. Such adaptations broaden the operational window of steam turbines and underscore their potential as a viable option in a low-carbon energy landscape.
Moreover, with the growing emphasis on energy storage solutions, steam turbines are often paired with thermal energy storage systems. Such configurations can provide grid stability and assist in meeting demand when renewable generation lags. By leveraging stored thermal energy, steam turbines can generate electricity more efficiently and ensure a continuous supply, further solidifying their role within future energy systems.
In conclusion, the future of steam turbines in energy production appears promising, characterized by their integration with renewable sources, advancements in efficiency, and their adaptability to cleaner fuel options. As the energy landscape continues to transform, steam turbines will remain pivotal in achieving sustainable energy goals and facilitating the transition towards a greener future.
Conclusion
In summary, steam turbines play an essential role in the modern energy landscape, serving as a backbone for electricity generation across various industries. The evolution of steam turbine technology has significantly improved efficiency and reliability, with advancements that accommodate higher pressures and temperatures, thus maximizing energy conversion from heat sources into mechanical energy. By harnessing thermal energy from steam, these turbines facilitate the efficient production of electricity, a crucial element in meeting the demands of growing populations and industrial applications.
Additionally, steam turbines are integral to the transition towards sustainable energy solutions. Their ability to operate in conjunction with renewable sources, such as biomass and concentrated solar power, highlights their versatility in contemporary energy frameworks. As global energy demands continue to rise, the importance of optimizing steam turbine systems becomes increasingly evident, particularly in achieving carbon reduction targets and enhancing overall system efficiency.
Ultimately, the complexity of steam turbine engineering merits deep appreciation as these remarkable machines are pivotal not only to energy production but also to addressing the challenges of climate change. Embracing innovations that enhance steam turbine performance ensures that this technology remains at the forefront of energy solutions. By understanding and investing in steam turbines, stakeholders can contribute to a sustainable energy future that meets the needs of both current and future generations.